MICROBIAL METABOLISM CONTD...
GLUCONEOGENESIS:
Gluconeogenesis, the formation of new glucose molecules from noncarbohydrate
precursors, occurs primarily in the liver. Precursor molecules include lactate, pyruvate,
glycerol, and certain -keto acids (molecules derived from amino acids).
Under certain conditions (i.e., metabolic acidosis or starvation) the kidney can
make small amounts of new glucose. Between meals adequate blood glucose
levels are maintained by the hydrolysis of liver glycogen. When liver glycogen
is depleted (e.g., owing to prolonged fasting or vigorous exercise), the gluconeogenesis
pathway provides the body with adequate glucose. Brain and red blood
cells rely exclusively on glucose as their energy source.
Gluconeogenesis Reactions:
The reaction sequence in gluconeogenesis is largely the reverse of glycolysis.
Recall, however, that three glycolytic reactions (the reactions catalyzed by
hexokinase, PFK-1, and pyruvate kinase) are irreversible. In gluconeogenesis,
alternate reactions catalyzed by different enzymes are used to bypass these obstacles.
Following are steps of this reaction:
Pyruvate + CO2 + H2O in presence of enzyme pyruvate carboxylase form oxaloacetate .
The transfer of CO2 to form the product OAA(Oxaloacetate) is mediated by the coenzyme
biotin (p. xxx), which is covalently bound within the enzyme’s active site. OAA is then decarboxylated and phosphorylated by PEP carboxykinase
in a reaction driven by the hydrolysis of guanosine triphosphate (GTP). PEP carboxykinase is found within the mitochondria of some species and
in the cytoplasm of others. In humans this enzymatic activity is found in
both compartments. Because the inner mitochondrial membrane is
impermeable to OAA, cells that lack mitochondrial PEP carboxykinase
transfer OAA into the cytoplasm by using the malate shuttle. In this
process, OAA is converted into malate by mitochondrial malate dehydrogenase.
After the transport of malate across mitochondrial membrane, the
reverse reaction (to form OAA and NADH) is catalyzed by cytoplasmic
malate dehydrogenase. The malate shuttle allows gluconeogenesis to continue
because it provides the NADH required for the reaction catalyzed
by glyceraldehyde-3-phosphate dehydrogenase.
2. Conversion of fructose-1,6-bisphosphate to fructose-6-phosphate: The irreversible PFK-1–catalyzed reaction in glycolysis is bypassed by
fructose-1,6-bisphosphatase.
3.) Formation of glucose from glucose-6-phosphate: Glucose-6-phosphatase,
found only in liver and kidney, catalyzes the irreversible hydrolysis of glucose-
6-phosphate to form glucose and Pi. Glucose is subsequently released
into the blood.
Each of the foregoing reactions is matched by an opposing irreversible
reaction in glycolysis. Each set of such paired reactions is referred to as
a substrate cycle. Because they are coordinately regulated (an activator
of the enzyme catalyzing the forward reaction serves as an inhibitor of the
enzyme catalyzing the reverse reaction), very little energy is wasted, even
though both enzymes may be operating at some level at the same time.
Flux control (regulation of the flow of substrate and removal of product)
is more effective if transient accumulation of product is funneled back
through the cycle. The catalytic velocity of the forward enzyme
will remain high if the concentration of the substrate is maximized. The
gain in catalytic efficiency more than makes up for the small energy loss
in recycling the product.
Gluconeogenesis is an energy-consuming process. Instead of generating ATP (as in glycolysis), gluconeogenesis requires the hydrolysis of six high energy
phosphate bonds.
Gluconeogenesis Substrates:
As previously mentioned, several metabolites are gluconeogenic precursors. Three
of the most important substrates are described briefly.
Lactate is released by red blood cells and other cells that lack mitochondria or
have low oxygen concentrations. In the Cori cycle, lactate is released by skeletal muscle
during exercise . After lactate is transferred to the liver, it is reconverted
to pyruvate by lactate dehydrogenase and then to glucose by gluconeogenesis.
Glycerol, a product of fat metabolism in adipose tissue, is transported to the
liver in the blood and then converted to glycerol-3-phosphate by glycerol kinase.
Oxidation of glycerol-3-phosphate to form DHAP occurs when cytoplasm NAD concentration is relatively high.
Of all the amino acids that can be converted to glycolytic intermediates (molecules
referred to as glucogenic), alanine is perhaps the most important. When
exercising muscle produces large quantities of pyruvate, some of these molecules
are converted to alanine by a transamination reaction involving glutamate.
After it has been transported to the liver, alanine is reconverted to pyruvate and
then to glucose. The glucose-alanine cycle serves several purposes.
In addition to its role in recycling -keto acids between muscle and liver, the
glucose-alanine cycle is a mechanism for transporting amino nitrogen to the
liver. In -keto acids, sometimes referred to as carbon skeletons, a carbonyl
group is directly attached to the carboxyl group. Once alanine reaches the liver,
it is reconverted to pyruvate. The amino nitrogen is then incorporated into urea
or transferred to other -keto acids to restore the amino acid balance in the liver.
Fig: Cori Cycle
Fig: Glucose-Alanine Cycle
Gluconeogenesis Regulation:
As with other metabolic pathways, the rate of gluconeogenesis is affected primarily
by substrate availability, allosteric effectors, and hormones. Not surprisingly,
gluconeogenesis is stimulated by high concentrations of lactate, glycerol,
and amino acids. A high-fat diet, starvation, and prolonged fasting make large
quantities of these molecules available.
The four key enzymes in gluconeogenesis (pyruvate carboxylase, PEP carboxykinase,
fructose-1,6-bisphosphatase, and glucose-6-phosphatase) are affected
to varying degrees by allosteric modulators. For example, fructose-1,6-bisphosphatase
is activated by citrate and inhibited by AMP and fructose-2,6-bisphosphate.
Acetyl-CoA activates pyruvate carboxylase. (The concentration of
acetyl-CoA, a product of fatty acid degradation, is especially high during starvation.)
As with other biochemical pathways, hormones affect gluconeogenesis by
altering the concentrations of allosteric effectors and the key rate-determining
enzymes. As mentioned previously, glucagon depresses the synthesis of fructose-
2,6-bisphosphate, which releases the inhibition of fructose-1,6-bisphosphatase,
and inactivates the glycolytic enzyme pyruvate kinase. Hormones also influence
gluconeogenesis by altering enzyme synthesis. For example, the synthesis of gluconeogenic
enzymes is stimulated by cortisol, a steroid hormone produced in
the cortex of the adrenal gland that facilitates the body’s adaptation to stressful
situations. Finally, insulin action leads to the synthesis of new molecules of glucokinase,
PFK-1 (SREBP1c-induced), and PFK-2 (glycolysis favored). Insulin
also depresses the synthesis (also via SREBP1c) of PEP carboxykinase, fructose-
1,6-bisphosphatase, and glucose-6-phosphatase. Glucagon action leads to the
synthesis of additional molecules of PEP carboxykinase, fructose-1,6-
bisphosphatase, and glucose-6-phosphatase (gluconeogenesis favored).
The hormones that regulate glycolyses and gluconeogenesis alter the phosphorylation
state of certain target proteins in the liver cell, which in turn modifies
gene expression. The key point to remember is that insulin and glucagon have
opposing effects on carbohydrate metabolism. The direction of metabolite flux, (i.e.,
whether either glycolysis or gluconeogenesis is active) is largely determined by
the ratio of insulin to glucagon. After a carbohydrate meal, the insulin/glucagon
ratio is high and glycolysis in the liver predominates over gluconeogenesis. After
a period of fasting or following a high-fat, low-carbohydrate meal, the
insulin/glucagon ratio is low and gluconeogenesis in the liver predominates over
glycolysis. The availability of ATP is the second important regulator in the reciprocal
control of glycolysis and gluconeogenesis in that high levels of AMP, the
low-energy hydrolysis product of ATP, increase the flux through glycolysis at
the expense of gluconeogenesis, and low levels of AMP increase the flux through
gluconeogenesis at the expense of glycolysis. Although control at the PFK-1/fructose-
1,6-bisphosphatase cycle would appear to be sufficient for this pathway, control
at the pyruvate kinase step is key because it permits the maximal retention of PEP,
a molecule with a very high phosphate transfer potential.
Gluconeogenesis, the formation of new glucose molecules from noncarbohydrate
precursors, occurs primarily in the liver. Precursor molecules include lactate, pyruvate,
glycerol, and certain -keto acids (molecules derived from amino acids).
Under certain conditions (i.e., metabolic acidosis or starvation) the kidney can
make small amounts of new glucose. Between meals adequate blood glucose
levels are maintained by the hydrolysis of liver glycogen. When liver glycogen
is depleted (e.g., owing to prolonged fasting or vigorous exercise), the gluconeogenesis
pathway provides the body with adequate glucose. Brain and red blood
cells rely exclusively on glucose as their energy source.
Gluconeogenesis Reactions:
The reaction sequence in gluconeogenesis is largely the reverse of glycolysis.
Recall, however, that three glycolytic reactions (the reactions catalyzed by
hexokinase, PFK-1, and pyruvate kinase) are irreversible. In gluconeogenesis,
alternate reactions catalyzed by different enzymes are used to bypass these obstacles.
Following are steps of this reaction:
1.) Synthesis of PEP: PEP synthesis from pyruvate requires two enzymes:
pyruvate carboxylase and PEP carboxykinase. Pyruvate carboxylase, found within mitochondria converts pyruvate into oxaloacetate.Pyruvate + CO2 + H2O in presence of enzyme pyruvate carboxylase form oxaloacetate .
The transfer of CO2 to form the product OAA(Oxaloacetate) is mediated by the coenzyme
biotin (p. xxx), which is covalently bound within the enzyme’s active site. OAA is then decarboxylated and phosphorylated by PEP carboxykinase
in a reaction driven by the hydrolysis of guanosine triphosphate (GTP). PEP carboxykinase is found within the mitochondria of some species and
in the cytoplasm of others. In humans this enzymatic activity is found in
both compartments. Because the inner mitochondrial membrane is
impermeable to OAA, cells that lack mitochondrial PEP carboxykinase
transfer OAA into the cytoplasm by using the malate shuttle. In this
process, OAA is converted into malate by mitochondrial malate dehydrogenase.
After the transport of malate across mitochondrial membrane, the
reverse reaction (to form OAA and NADH) is catalyzed by cytoplasmic
malate dehydrogenase. The malate shuttle allows gluconeogenesis to continue
because it provides the NADH required for the reaction catalyzed
by glyceraldehyde-3-phosphate dehydrogenase.
2. Conversion of fructose-1,6-bisphosphate to fructose-6-phosphate: The irreversible PFK-1–catalyzed reaction in glycolysis is bypassed by
fructose-1,6-bisphosphatase.
3.) Formation of glucose from glucose-6-phosphate: Glucose-6-phosphatase,
found only in liver and kidney, catalyzes the irreversible hydrolysis of glucose-
6-phosphate to form glucose and Pi. Glucose is subsequently released
into the blood.
Each of the foregoing reactions is matched by an opposing irreversible
reaction in glycolysis. Each set of such paired reactions is referred to as
a substrate cycle. Because they are coordinately regulated (an activator
of the enzyme catalyzing the forward reaction serves as an inhibitor of the
enzyme catalyzing the reverse reaction), very little energy is wasted, even
though both enzymes may be operating at some level at the same time.
Flux control (regulation of the flow of substrate and removal of product)
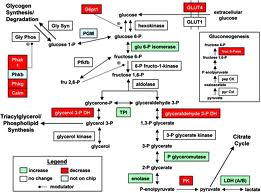
through the cycle. The catalytic velocity of the forward enzyme
will remain high if the concentration of the substrate is maximized. The
gain in catalytic efficiency more than makes up for the small energy loss
in recycling the product.
Gluconeogenesis is an energy-consuming process. Instead of generating ATP (as in glycolysis), gluconeogenesis requires the hydrolysis of six high energy
phosphate bonds.
Gluconeogenesis Substrates:
As previously mentioned, several metabolites are gluconeogenic precursors. Three
of the most important substrates are described briefly.
Lactate is released by red blood cells and other cells that lack mitochondria or
have low oxygen concentrations. In the Cori cycle, lactate is released by skeletal muscle
during exercise . After lactate is transferred to the liver, it is reconverted
to pyruvate by lactate dehydrogenase and then to glucose by gluconeogenesis.
Glycerol, a product of fat metabolism in adipose tissue, is transported to the
liver in the blood and then converted to glycerol-3-phosphate by glycerol kinase.
Oxidation of glycerol-3-phosphate to form DHAP occurs when cytoplasm NAD concentration is relatively high.
Of all the amino acids that can be converted to glycolytic intermediates (molecules
referred to as glucogenic), alanine is perhaps the most important. When
exercising muscle produces large quantities of pyruvate, some of these molecules
are converted to alanine by a transamination reaction involving glutamate.
After it has been transported to the liver, alanine is reconverted to pyruvate and
then to glucose. The glucose-alanine cycle serves several purposes.
In addition to its role in recycling -keto acids between muscle and liver, the
glucose-alanine cycle is a mechanism for transporting amino nitrogen to the
liver. In -keto acids, sometimes referred to as carbon skeletons, a carbonyl
group is directly attached to the carboxyl group. Once alanine reaches the liver,
it is reconverted to pyruvate. The amino nitrogen is then incorporated into urea
or transferred to other -keto acids to restore the amino acid balance in the liver.
Fig: Cori Cycle
Fig: Glucose-Alanine Cycle
Gluconeogenesis Regulation:
As with other metabolic pathways, the rate of gluconeogenesis is affected primarily
by substrate availability, allosteric effectors, and hormones. Not surprisingly,
gluconeogenesis is stimulated by high concentrations of lactate, glycerol,
and amino acids. A high-fat diet, starvation, and prolonged fasting make large
quantities of these molecules available.
The four key enzymes in gluconeogenesis (pyruvate carboxylase, PEP carboxykinase,
fructose-1,6-bisphosphatase, and glucose-6-phosphatase) are affected
to varying degrees by allosteric modulators. For example, fructose-1,6-bisphosphatase
is activated by citrate and inhibited by AMP and fructose-2,6-bisphosphate.
Acetyl-CoA activates pyruvate carboxylase. (The concentration of
acetyl-CoA, a product of fatty acid degradation, is especially high during starvation.)
As with other biochemical pathways, hormones affect gluconeogenesis by
altering the concentrations of allosteric effectors and the key rate-determining
enzymes. As mentioned previously, glucagon depresses the synthesis of fructose-
2,6-bisphosphate, which releases the inhibition of fructose-1,6-bisphosphatase,
and inactivates the glycolytic enzyme pyruvate kinase. Hormones also influence
gluconeogenesis by altering enzyme synthesis. For example, the synthesis of gluconeogenic
enzymes is stimulated by cortisol, a steroid hormone produced in
the cortex of the adrenal gland that facilitates the body’s adaptation to stressful
situations. Finally, insulin action leads to the synthesis of new molecules of glucokinase,
PFK-1 (SREBP1c-induced), and PFK-2 (glycolysis favored). Insulin
also depresses the synthesis (also via SREBP1c) of PEP carboxykinase, fructose-
1,6-bisphosphatase, and glucose-6-phosphatase. Glucagon action leads to the
synthesis of additional molecules of PEP carboxykinase, fructose-1,6-
bisphosphatase, and glucose-6-phosphatase (gluconeogenesis favored).
The hormones that regulate glycolyses and gluconeogenesis alter the phosphorylation
state of certain target proteins in the liver cell, which in turn modifies
gene expression. The key point to remember is that insulin and glucagon have
opposing effects on carbohydrate metabolism. The direction of metabolite flux, (i.e.,
whether either glycolysis or gluconeogenesis is active) is largely determined by
the ratio of insulin to glucagon. After a carbohydrate meal, the insulin/glucagon
ratio is high and glycolysis in the liver predominates over gluconeogenesis. After
a period of fasting or following a high-fat, low-carbohydrate meal, the
insulin/glucagon ratio is low and gluconeogenesis in the liver predominates over
glycolysis. The availability of ATP is the second important regulator in the reciprocal
control of glycolysis and gluconeogenesis in that high levels of AMP, the
low-energy hydrolysis product of ATP, increase the flux through glycolysis at
the expense of gluconeogenesis, and low levels of AMP increase the flux through
gluconeogenesis at the expense of glycolysis. Although control at the PFK-1/fructose-
1,6-bisphosphatase cycle would appear to be sufficient for this pathway, control
at the pyruvate kinase step is key because it permits the maximal retention of PEP,
a molecule with a very high phosphate transfer potential.
Comments